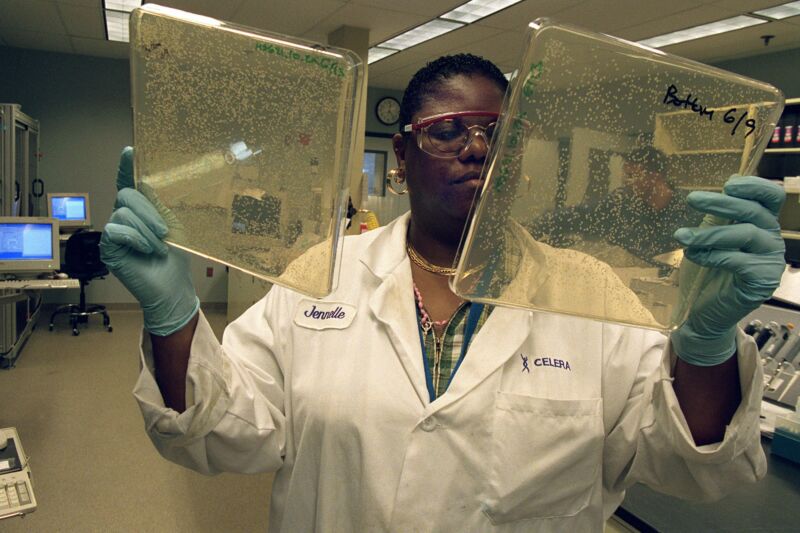
Many of the fundamental features of life don't necessarily have to be the way they are. Chance plays a major role in evolution, and there are always alternate paths that were never explored, simply because whatever evolved previously happened to be good enough. One instance of this idea is the genetic code, which converts the information carried by our DNA into the specific sequence of amino acids that form proteins. There are scores of potential amino acids, many of which can form spontaneously, but most life uses a genetic code that relies on just 20 of them.
Over the past couple of decades, scientists have shown that it doesn't have to be that way. If you supply bacteria with the right enzyme and an alternative amino acid, they can use it. But bacteria won't use the enzyme and amino acid very efficiently, as all the existing genetic code slots are already in use.
In a new work, researchers have managed to edit bacteria's genetic code to free up a few new slots. They then filled those slots with unnatural amino acids, allowing the bacteria to produce proteins that would never be found in nature. One side effect of the reprogramming? No viruses could replicate in the modified bacteria.
Lost in translation
The genetic code handles translation, during which the information encoded in DNA is made into a functional protein. Key to this process is a group of small RNA molecules called transfer RNAs (or tRNAs). Transfer RNAs have a small, three-base segment that can be matched through base pairing, with information carried by DNA. RNAs can also be chemically linked to a specific amino acid in a process catalyzed by particular enzymes.
That combination—three specific bases paired with a particular amino acid—is the key to translation, i.e., to matching the bases of DNA with a specific amino acid.
A three-base code and four possible bases (A, T, C, and G) yield 64 possible three-base combinations, called codons. Three of those codons signal for translation to be stopped when the end of the protein-coding sequence is reached. That leaves 61 codons for only 20 amino acids. As a result, some amino acids are encoded by two, four, or even six different codons.
That redundancy in the code is what the research team—based in Cambridge, UK—targeted. A few years ago, the researchers edited the entire E. coli genome so that some of the redundant codons were freed up. The research team edited all instances of one of the three stop codons into one of the others so that there were no longer any instances of it in the entire genome. Instead of being used for something, the codon was freed up to be redefined.
The researchers did similar experiments with the codons for the amino acid serine. Instead of leaving six codons that say "serine," the team edited the total down to just four by changing every instance of the two they targeted to a different serine codon.
(That may sound simple, but even a small genome like E. coli's has thousands of each of these codons scattered through millions of base pairs. Editing the genetic code is an impressive technical achievement on its own.)
Tolerating change
While the bacteria didn't use the three edited codons, they still could. All the pieces needed to use the codons—the transfer RNAs, the enzymes that attach amino acids to them, etc.—were still present. For reasons that aren't entirely clear, the modified bacteria weren't especially healthy and grew at a slower pace than their unedited source.
For their follow-up work, the researchers evolved the strain to tolerate the modified genetic code better. They exposed the bacteria to mutagens and then grew lots of samples using an automated system that identified when a sample was growing well and kept supplying the sample with fresh food. (Fast-growing bacteria turn whatever they're grown in cloudy, allowing them to be identified.) After a couple of rounds of mutation, near-normal growth was restored.
At that point, the researchers went back and deleted the genes for the transfer RNAs and enzymes that allowed their three edited codons to work. With those changes made, it wasn't that the codons were no longer being used—they could no longer be used.
Again, this issue slowed down the growth of the bacteria, although it's not clear why—either some of the deleted genes have other functions or there were codon instances the researchers missed in editing. Regardless, they mutated the bacteria again and selected a strain in which much of the growth had been restored. By the time everything was done, the scientists had a strain that grew about half as well as a normal E. coli. They also had three completely unused codons.
(As an aside, the team also obtained a genome sequence of this final strain to see what mutations had occurred during this process. Although numerous differences were identified, none were obviously associated with the ability to grow with a modified genetic code. The lab has undoubtedly since assigned a few grad students to figure out that conundrum.)
New code, who dis?
To confirm that the three unused codons were nonfunctional, the researchers infected them with viruses. The proteins encoded by these viruses normally include the unused codons, so this method provides a test of whether the codons' use was truly eliminated.
The bacteria passed the test. No viruses could grow in the codons, even when a mixture of five different viruses were thrown in the culture at the same time. It was clear that in this strain, these codons simply could not be used.
That's what the researchers wanted in the first place (it's fair to say they didn't set out to make virus-resistant bacteria). Now they could start using the three codons for amino acids that aren't naturally used by life on Earth.
The researchers supplied the bacteria with some non-native amino acids, along with the genes for a transfer RNA to attach the amino acids to and an enzyme that would do the attaching. They then started inserting the gene for a nonbacterial protein that could only be translated by using the codons they had redefined and confirmed that the protein was made and that it incorporated these non-natural amino acids. The team even made a version that incorporated three different artificial amino acids, showing that they truly had expanded the genetic code.
The researchers were also able to make strains that used a different set of three artificial amino acids. So it's possible to make a large collection of strains, each specialized to use a different set of artificial amino acids.
Interesting polymer chemistry
The authors didn't go on to demonstrate anything practical, but there are plenty of potential uses for the research. Artificial amino acids can potentially catalyze reactions that aren't possible or efficient with the normal set of 20. And we don't have to necessarily design an enzyme that incorporates the new amino acids; instead, we can simply try to evolve the function in strains with an expanded genetic code.
There's also the possibility for some interesting polymer chemistry. In the chemical reactions that form most polymers, we typically use only a single type of subunit to build the polymer, since you can't control what links with what. But proteins let you build a polymer chain with complete control of the order of each subunit because you can specify the order of amino acids. With an expanded genetic code, we can potentially get molecule-level control over the construction of polymers.
Science, 2021. DOI: 10.1126/science.abg3029 (About DOIs).
Recommended Comments
There are no comments to display.
Join the conversation
You can post now and register later. If you have an account, sign in now to post with your account.
Note: Your post will require moderator approval before it will be visible.